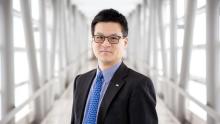
Humans are complex beings, but our inner workings operate using basic biological processes shaped and repurposed over hundreds of millions of years of evolution. By providing critical insights into these processes, decades of research using model organisms has been central to progress toward understanding and treating a range of human diseases – including neurologic, psychiatric, and neurodegenerative conditions.
Neuroscience knowledge gained through research helped by the relative simplicity of model organisms has generated many principles, or “ground truths,” about the biology of the brain. From these ground truths we can infer function. One profound example: in terms of their electrical properties, neurons basically work the same in all living organisms. We learned about the underlying principles of neuronal excitability more than 70 years ago through the experiments of Hodgkin and Huxley using outsized (and easy to see) nerve cells of the giant squid. Nervous systems simpler than our own give us the chance to reveal and model new ideas, principles, and tools before applying them to more complex brains that we know far less about.
Through ongoing discovery and the application of new tools, the NIH BRAIN Initiative continues to uncover and build on previous discoveries from research using a range of model systems. One such example is a recently published finding about some of the physiological mechanisms underlying sensory perception and behavior from Dr. Matthieu Louis of the University of California, Santa Barbara. In this BRAIN-funded work, Louis and his team used larval Drosophila (juvenile fruit flies) to reveal how brain circuits manage sensory overload and allow the animal to complete a task at hand. Using this simple yet nimble model system in combination with powerful genetic, optogenetic, behavioral, and computational tools, Louis and his colleagues revealed that the fly larva’s olfactory system employs a mechanism known as “depolarization block.” This process stops the flow of electrical signals through voltage-gated ion channels in sensory neurons when an odor is strong (for example, when the larva gets very close to a ripe banana that’s emitting an attractive smell). By silencing these “saturated” or overloaded sensory neurons, the system can now respond to other neurons that are less sensitive to the odor (and therefore not blocked). In this way, depolarization block allows the system as a whole to remain responsive to an attractive odor even as it approaches the odor’s source, where the concentrations will be very high. This in turn allows the animal to complete the important task of navigating toward the food.
Are these observations relevant only to a fruit fly larva searching for food? Probably not. Several decades ago working with newts, meticulous measurements by Drs. Takahashi Kurahashi, Graeme Lowe, and Geoffrey Gold showed that very high concentrations of odors suppressed the responses of amphibian olfactory sensory neurons. Subsequent work went on to show that voltage-gated sodium channels (like those blocked in fruit fly larva) were targets of the newts’ odor-induced suppression. For me, it’s breathtaking that depolarization block can be found in the olfactory sensory systems of invertebrates (like flies) and vertebrates (like newts) spanning hundreds of millions of years of evolution. And since the initial discovery of depolarization block in newts, the evolution in experimental and computational approaches has allowed – using the fruit fly larva – a deeper understanding of the mechanisms behind this phenomenon and how it might be used to influence sensory-guided behaviors. This is also not likely the end of the story, because depolarization block has been linked to debilitating conditions that affect humans, such as migraines and epileptic seizures.
BRAIN-funded research adds to a growing list of fundamental principles identified through research with model organisms like yeast, flies, worms, and mice. For example, work in the roundworm C. elegans has taught us how nervous systems develop and synapses form, revealing that the process – including the genes and proteins involved – appears to be remarkably similar across organisms. The roundworm has been transformative for neuroscience in part because we have complete catalogs of all the animal’s cells, genes, as well as a comprehensive wiring diagram of its nervous system. And just recently, a detailed wiring diagram of a complete larval fruit fly brain was revealed, work that was supported in part by the BRAIN Initiative. New wiring diagrams in ever more complex animals (with more yet to come from new transformative projects such as the BRAIN Initiative Connectivity across Scales [BRAIN CONNECTS] project) will continue to firm up what we know about the organization of brain circuits and how they process information.
Beyond providing windows into the marvelous beauty and complexity of brains and nervous systems, discoveries made in model organisms also offer fertile territory for prototyping new tools, some of which may ultimately be applied for new therapies in humans. For example, since neurons operate in much the same way from squids to humans, BRAIN-funded research aiming to change the activity of neurons using light, or to deliver payloads to brain circuits using viruses, or to implant sensors to understand how hallucinogens affect the brain, may point to new treatment and prevention strategies for a variety of neurodegenerative and psychiatric disorders.
But the value of model organisms isn’t limited to cases where they can directly predict what happens in larger brains, including those in humans. It’s that systems simpler and more well-characterized than humans provide facile models for formulating, testing, and ultimately falsifying – or “truthifying” – hypotheses about how brain cells and circuits underlie complex behaviors in health and disease. Even when a model system is shown not to have a direct correlate in human brain biology, we learn something about how evolution has shaped a solution for an organism’s particular context. Deep understanding of biology’s rules through deconstruction and reconstruction of processes in systems simpler than humans should help us learn how to reprogram pathways and circuits to repair or restore function when needed. Insights enabled by new tools and grounded in evolutionary principles comprise a cornerstone of the BRAIN Initiative, which is aimed at accelerating discovery in search of new knowledge and new cures.
With respect and gratitude,